by Dirk Dusharme
Developing test equipment that
can inspect materials or assemblies without destroying or
disassembling them is of interest to most industries and
critical to many. Aircraft, nuclear facilities and pipeline
inspections would prove cost-prohibitive if it weren’t
for the array of nondestructive evaluation tools available
to those industries.
One key NDE tool is eddy current testing. In commercial
use since the 1950s, eddy current testing provides a versatile
method for the nondestructive evaluation of conductive materials
when access to the material is limited to one side or when
it’s necessary to detect flaws beneath the surface.
Unlike ultrasonic methods, this technology works even when
an air gap exists in the tested material, making it ideal
for nondestructive evaluation of laminated metallic materials.
This article discusses some of the newer eddy current
technologies and their implications for nondestructive evaluation,
particularly when the area under inspection has a large
surface or varying thickness.
If
you apply an alternating current to a wire wrapped around
a conductor (e.g., the wire in an eddy current probe), an
alternating electromagnetic field forms around the conductor.
Bring this field into proximity with another conductive
material--a pipe, pressure vessel, area of aircraft wing,
etc.--and an alternating electrical current will flow in
that portion of the material as well. This alternating current
causes its own secondary magnetic field that interacts with
(i.e., adds vectorially to) the primary field and results
in a perturbation to the field around the probe, as illustrated
on the right.
As long as there’s no change in the material or
its proximity to the probe, the perturbation remains constant
and can be measured. If while moving along the material
under test, the probe passes over a crack, a region of corrosion,
an overstressed area, a hole or some other anomaly, a subtle
change in the field occurs. This change can be measured
and compared to measurements on a similar known-good material
and the results quantified by the operator and the eddy
current instrumentation.
Because the response to the magnetic field depends upon
the material’s conductivity, magnetic permeability
and distance from the probe to the material’s conductive
surface, eddy current testers can gather information in
addition to the location of flaws or corrosion. For example,
they can provide information about the physical properties
of the material or the thickness of nonconductive coatings,
such as paint. If the material isn’t too thick, eddy
currents can also measure thickness.
Changing the frequency of the probe’s excitation
current changes the depth to which the probe can penetrate:
the lower the frequency, the deeper the measurement. Thus,
a low-frequency eddy current instrument can detect subsurface
defects or defects on the opposite side of the scanned material.
This makes eddy current testing ideal for detecting corrosion
or cracks in areas invisible from the surface. Often, measurements
are made at multiple frequencies in order to inspect the
material at multiple depths.
However, several issues hamper the conventional technology’s
productivity when the method is used to inspect large areas
of complex parts of varying thickness, such as aircraft
frames. First, distinguishing between defects and probe
liftoff--or mere structural changes such as plate separation,
edges and fasteners--requires multiple-frequency or swept-frequency
eddy current equipment, both of which involve more setup
time than single-frequency measurement. Second, measuring
a sample that has greatly varying thickness requires a different
setup for each thickness in order to obtain optimum defect
distinction and, for each change in structure, a new known-good
specimen must be manufactured for setup purposes. Third,
unlike many measurement technologies, conventional eddy
current inspection takes comparative rather than absolute
measurements and is highly dependent upon users having a
good understanding of the type of material under inspection
and the faults they expect to find. Therefore, it requires
some skill in both setup and interpreting results.
Advances in eddy current technology during the past five
years have addressed some of these problems.
When using conventional eddy current techniques to inspect
materials for flaws that might occur at varying depths,
inspectors must establish multiple excitation frequencies.
In commercial applications, these tests are typically done
in two ways: by taking multiple measurements at different
frequencies using a single-frequency instrument or by using
a multiple-frequency instrument. The former suffers from
obvious productivity issues: Before measuring, the user
must set up the probe and perform a calibration for each
frequency. With a multiple-frequency instrument, the technician
can select from a number of frequencies, which the probe
will use simultaneously in a single measurement. To a certain
extent with either method, users must have some understanding
of the material’s properties, the types of flaws expected
and the depth at which they expect flaws to occur to select
the appropriate frequencies.
A new technology, pulsed (or transient) eddy current,
uses a shaped waveform rather than a continuous sine wave
to excite the coil and generate an eddy current pulse in
the structure. Because a pulse represents the sum of sine
waves for a broad band of frequencies, one test pulse can
contain all the frequencies needed to perform the tests
at different depths, explains Marcus Johnson, associate
scientist with the Center for Nondestructive Evaluation
at Iowa State University. “The real advantage is that
pulsed eddy current is rich in low-frequency components
so you’re able to see near-surface and deeper flaws
at the same time,” he notes.
Iowa State’s pulsed eddy current probe is excited
by a pulse, which generates a broadband eddy current field
in the sample. The higher-frequency eddy current components
are generated more quickly within the material under test
and lead to a rapid, high-amplitude response, as seen by
the probe, says Johnson.
If you look at the pulsed eddy current response as a function
of time, the waveform’s earlier portion corresponds
to near-surface artifacts such as liftoff. The middle portion
corresponds to midsample artifacts such as internal cracks
or interlayer corrosion, and the tail end of the waveform
could indicate overall thickness or show bottom surface
artifacts such as corrosion, as illustrated below.
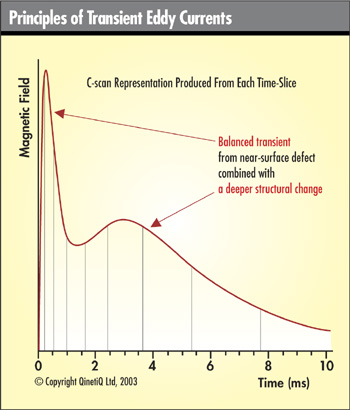
Pulsed eddy currents offer an advantage over multiple-frequency
probes because users don’t have to select the frequencies
at which to scan, says Robert A. Smith of the United Kingdom-based
QinetiQ Ltd.
“The basic difference is that transient eddy current
captures data covering all the frequencies in the bandwidth,
whereas multiple-frequency eddy current only captures certain
specific frequencies,” says Smith. “This means
that the optimum frequencies for any part of the structure
will always be available from any transient eddy current
scan. For a multiple-frequency scan, the optimum frequencies
would be determined in advance and will be different for
each different structure, requiring several scans over complex
structural changes.”
Pulsed eddy current technology offers more information
about structure and defects than multiple-frequency eddy
currents, adds Jesse Skramstad, president of NDT Solutions
Inc., the U.S. distributor of QinetiQ’s TRECSCAN transient
eddy current instrument. The probe is also much easier to
use, adds Skramstad. “The same probe and simple setup
can be used over a wide range of structural thickness, thus
speeding up the data acquisition process,” he notes.
This technology offers the following advantages, according
to Skramstad:
Because of the broadband characteristic of pulsed eddy current,
the full frequency range is captured in one pass.
Large areas of structure with multiple variations in thickness
can be scanned without the need for probe or setup changes.
The same setup can be used for different structures, which
are optimized during post-processing. This eliminates the
need for specimens representing the structures encountered,
says Smith. “Unlike traditional eddy current measurements,
pulsed eddy current doesn’t require the production
of multiple known-good specimens for setup or reference
purposes,” he says.
Compared to inductive coils, the use of Hall-effect sensors
(explained later in the article) as field detectors improves
the spatial resolution and detectability of deep defects.
Advanced post-processing analysis tools allow better data
analysis by using algorithms for liftoff compensation, edge
subtraction (i.e., edge correction), total thickness measurement,
plate separation effect elimination, time-slice viewing
and time-domain signal processing. All referencing to known-good
structure can be accomplished on the scan data itself.
Johnson cautions that with pulsed eddy current there are
some signal-to-noise issues to consider that don’t
exist with multiple-frequency instruments. In the latter
case, the instrument can lock onto the exact frequencies
being analyzed, eliminating the noise at all other frequencies.
Scanning
eddy current equipment can use an array of individual eddy
current probes in order to increase the area that can be
scanned in one pass. This has huge implications for industries
that require a lot of eddy current measurements, particularly
airframe inspection, in which eddy currents are used to
examine every fastener on an airframe’s surface looking
for minute cracks.
“Right now, every single fastener has to be looked
at with a probe using circular symmetry,” explains
Ray Rempt, technical fellow with Boeing Phantom Works in
Seattle. “It takes a minute or more per fastener if
you don’t use an array. You can inspect dozens of
fasteners per minute with a scanning array.” Rempt’s
work with Boeing involves developing scanning arrays of
about an inch wide, enough to span a row of fasteners on
a fuselage. He estimates that eddy current inspection accounts
for about 80 percent of airframe inspection for aircraft
depot operations.
“They cover a larger area in less inspection time,”
agrees Marc Grenier, product manager for R/D Tech, a Quebec-based
developer of ultrasonic and eddy current instrumentation.
“In a single pass, you get a better idea of the structure
of the fault. If you try to do this with a pencil probe,
it’s very difficult to get an idea of the size of
the flaw. With an array, you get a topographic representation
of the defect. So you get shape and depth in a single pass.”
A pencil probe could collect the same information, but it
would take so long to do that the method is usually avoided,
says Grenier. One of R/D Tech's array instruments is shown
above.
The other advantage of eddy current arrays is that they
can be shaped to fit specific geometries--hexagonal, square,
flat and even more complex shapes, such as turbine blades.
“Instead of using robotics with three, four or five
axes to rotate a part around a single probe, you could use
a robot with a single axis to simply move a part in and
out of an array,” says Grenier.
He stresses that eddy current arrays don’t provide
more resolution than would be obtainable with a pencil probe.
In fact, when developing arrays for a client, R/D Tech first
determines which type of standard probe is required for
the measurement task and then creates an array of those
specific probe types.
The number of probes in an array also depends on the task,
he says. “For manual testing in aerospace, lap joint
inspection, looking for corrosion under aluminum or determining
cracks around fasteners, we might typically have 32 elements
in the array,” explains Grenier. “When you go
to tube inspection, our standard X-Probe has 48 elements.”
For more complex applications, the company has supplied
arrays with up to 200 elements.
The key component in any eddy current system is the sensor
used to measure the magnetic field. Traditionally these
have been inductive coils. Although in use for decades,
coils do have a few drawbacks. The coils’ physical
makeup limits them in resolution and measurement depth,
the latter being dependent upon a coil’s insensitivity
to low-frequency signals. The coils’ size also limits
the size of arrays that can be constructed from them.
To address the latter problem, a team from GE Global Research,
GE Aircraft Engines and GE Inspection Technologies has developed
a new product: coils constructed on a thin, flexible plastic
substrate. Instead of wire wound around a core, these flexible
sensors use metal lines (or traces) deposited on a flexible
plastic-like material, similar to a flexible printed circuit
board. They can be designed with single or multiple coils.
“This technology is conformable; we can shape it
to fit any part,” says Mike Bernstein of GE Inspection
Technologies. One application involves measuring dovetail
slots on jet engines, as illustrated on page 26. Using flexible
eddy current sensors in an automated system has allowed
GE to reduce the time for this task, which once took 14
hours, down to about 45 minutes.
A concern that immediately comes to mind is the durability
of these probes in a production environment. “The
arrays have now been in use in our manufacturing operations
for several years,” says Bernstein. “The repeatability
is incredible and the durability is excellent. Although
no probe has an infinite life, the GE array probe is far
more cost-effective than single-element applications. These
don’t last as long [as conventional probes], but we
get a tremendous number of parts inspected per probe.”
The probes are also easily replaced should they wear out,
Bernstein notes, and likens the chore to replacing a razor
blade.
GE has also developed accompanying integrated systems
and software to help deal with typical eddy current measurement
issues, such as automation, liftoff and edge effects.
Another flexible and conformable technology has been developed
by Jentek Sensors Inc. of Waltham, Massachusetts. The company’s
meandering wire magnetometer sensor consists of a primary
winding of a specific shape--typically laid out in the shape
of a square wave pattern with specific distances between
each of the waves. Around this meandering pattern are multiple
secondary windings. As with GE’s flexible coil, the
MWM is constructed as a thin flexible circuit board.
According to articles published by the company, the sensors
have high spatial resolution and reproducibility, allowing
their response to be accurately modeled--cutting down on
the amount of calibration required. Their thinness allows
them to be permanently installed in critical areas for continuous
monitoring of fatigue, according to some sources. We were
unable to contact Jentek to determine if the product has
yet been used in this fashion.
Both wound coils and flexible coils measure magnetic flux
indirectly; that is, they measure the current created in
the coil when its magnetic fields change. This indirect
rate-of-change measurement has limitations when trying to
probe deeper into a material where only low frequencies
can penetrate.
Two new technologies, Hall-effect devices and giant magnetoresistive
sensors, address this issue by directly measuring magnetic
flux. Both offer better spatial resolution at increasing
depth. With a coil you must sacrifice resolution to increase
its sensitivity at lower frequencies.
“The primary advantage of the GMR is that it detects
the field directly,” explains Rempt. “You’re
less dependent on frequency, so you can see deeper better.”
Believing them to be more stable and sensitive than Hall-effect
devices, Boeing is considering GMR to replace anisotropic
magnetoresistive sensors, a similar technology it now uses
for its eddy current probes.
Advances in new Hall-effect devices have somewhat addressed
Boeing’s concern, argues Johnson. Although he agrees
that their sensitivity might be an issue in conventional
eddy current measurements, he maintains that it’s
less of an issue where pulsed eddy current is concerned
because those systems can operate at much higher currents
and produce stronger fields within the sensitivity range
of Hall-effect probes.
Part of the attractiveness of Hall-effect sensors for
Iowa State, QinetiQ and others is that the devices have
been mass produced for decades and lend themselves to eddy
current sensor applications in terms of cost and functionality.
QinetiQ uses Hall-effect sensors in an array constructed
of a single rectangular coil with multiple Hall-effect sensors.
“Hall arrays offer additional benefits of good near-surface
resolution due to the small Hall-effect sensor size coupled
with the deep penetration provided by the large coil, and
hence the large magnetic field,” explains Smith, who
recently presented such an array for use with transient
eddy currents.
Eddy current measurement is a mature technology for the
nondestructive evaluation of conductive materials. It can
inspect for surface, subsurface and rear surface flaws in
thin materials. Recent advances in the technology include
pulsed eddy current, eddy current arrays, flexible probes
and new probe technologies such as Hall-effect and GMR sensors.
All of these advancements in eddy current help improve its
use with materials of varying degrees of thickness, increase
the speed at which measurements can be taken, allow eddy
current probes to reach previously unreachable areas and
improve eddy current’s depth of penetration and spatial
resolution.
Dirk Dusharme is Quality Digest’s technology
editor.
The author would like to thank the following individuals
for their assistance in this article: Marcus Johnson, the
Center for Nondestructive Evaluation at Iowa State University;
Ray Rempt, Boeing Phantom Works in Seattle; Mike Bernstein,
GE Inspection Technologies in Cincinnati; and Marc Grenier,
R/D Tech in Quebec.
For extensive and clearly presented information on
eddy current and other NDE technologies, visit www.ndt-ed.org,
an educational Web site developed by the Collaboration for
Nondestructive Testing and supported by academic and industrial
sponsors.
|